Dr. Guigen Li
Title: Paul W. Horn Distinguished Professor
Education: Ph.D., University of Arizona (Victor J. Hruby), 1995
Postdoctoral research, The Scripps Research Institute (K. Barry Sharpless, 2001 and
2022 Nobel Laureate in Chemistry), 1995-97.
Played a key role in the discovery of asymmetric aminohydroxylation reaction (Sharpless
AA).
Associate Editor, Molecules (Basel, Switzerland)
Associate Editor, Frontiers in Chemistry (Lausanne, Switzerland)
Associate Editor, Research (Washington DC)
Research Area: Organic, Chirality, Medicinal and Bioorganic Chemistry
Office: Chemistry 300-A
Phone: 806-834-8755
Email: guigen.li@ttu.edu
Prof Li and his coworkers have achieved over 370 publications (h-index = 61). His group is currently conducting the following research.
1. Multi-Layer Folding Chirality
(a). Li G. et al, Research, 2022, 2021: 9847949 (a Science partner journal of, by AAAS), https://doi.org/10.34133/2022/9847949
(b). Li G. et al, Chem. Eur. J., 2022, e202104102,https://doi.org/10.1002/chem.202104102 (VIP & Front Cover Art)
(c). Li G. et al, Research, 2021, 2021: 3565791 (a Science partner journal of, by AAAS), https://spj.sciencemag.org/journals/research/aip/3565791/
(d). Li G. et al, Natl Sc Rev, 2020, https://doi.org/10.1093/nsr/nwz203; https://academic.oup.com/nsr/article/8/1/nwaa205/5899765
(e). Li G. et al, Research, 2019, 2019: 6717104 (a Science sister journal of, by AAAS), https://doi.org/10.34133/2019/6717104
Recently, Li group has discovered new multi-layer 3D chirality. The chirality is characterized by sandwich arrangement: it is composed of three almost parallel planes, with the middle plane as the central layer, one layer at the top and the other layer at the bottom of the central plane (Figures 1 & 2). The upper and lower layers are interdependent and mutually restricted to avoid the possible racemization of mirror isomers. The multi-layer 3D chiral molecules and their precursors exhibit characteristics rarely seen in normal small organic molecules: they form long bars or nest-like solids, exhibit multi-colored fluorescence and AIE, and some of them exhibit extremely strong optical rotation (Figure 2).
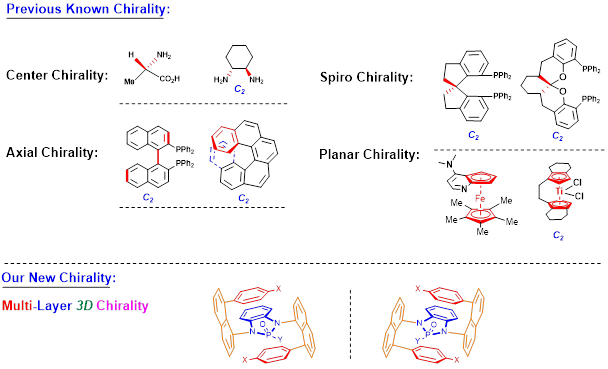
Figure 1. Well-known and new chirality types
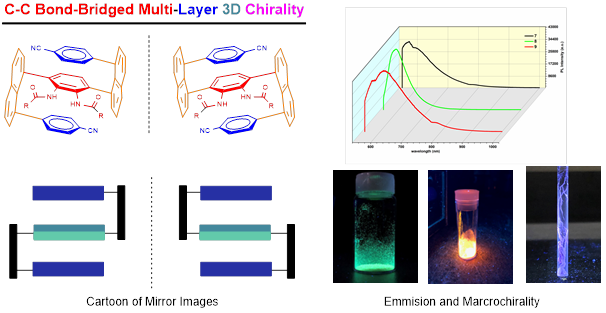
Figure 2. Photo properties of multi-layer 3D molecules
2. GAP Chemistry and Reagents
(Org. Biomol. Chem., 2017, 15, 1718; J. Am. Chem. Soc., 2020, 142, 8910)
Group-Assisted-Purification (GAP) chemistry is to introduce specific functional groups into the substrates for synthetic reactions so that the products in the subsequent purification process does not need to go through column chromatography or recrystallization, only a simple washing is needed to afford pure products (Scheme 1 & Figure 3). It is the first concept combining four chemical and physical aspects together: reaction, reagents, separation, and purification. It requires efficient control of solubility, stability, reactivity and selectivity for a broad scope of reactions and synthesis.
GAP chemistry can convert organic compounds for sticky oils into solids. When GAP chemistry is utilized for peptide synthesis, it can increase chemical yields (GAsyn chemistry-group-assisted synthesis), and enables Fmoc protection group to be usable for solution-phase peptide synthesis. GAP chemistry has shown success in recovering/recycling catalysts for re-use, including organo and organometallic catalysts (in collaboration with Profs Zhang and Findlater, Scheme 2).
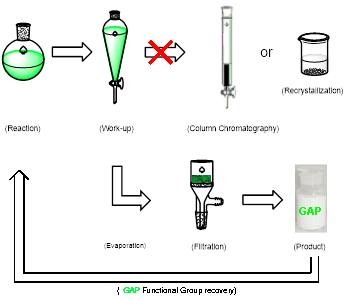
Figure 3. GAP chemistry description
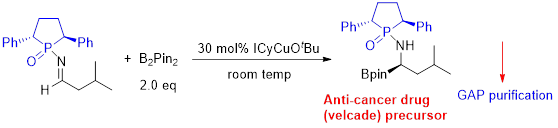
Scheme 1. GAP chemistry application
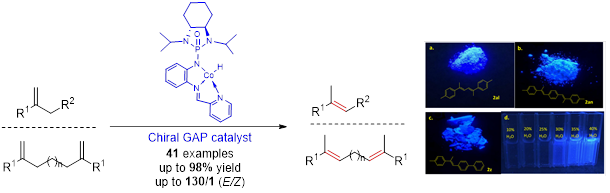
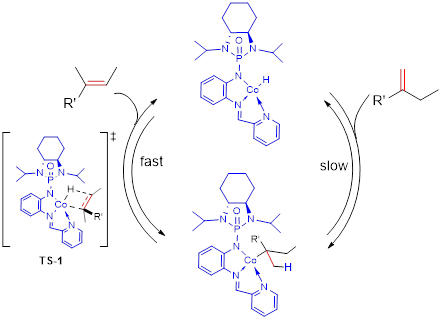
Scheme 2. GAP catalyst of recycling for re-use
3. Asymmetric Catalytic Approach to Multi-Layer 3D Chirality
(Chem. Eur. J., 2021, 27, 8013-8020, https://doi.org/10.1002/chem.202100700) (VIP & Front Cover Art)
Asymmetric one C-C bond formation controls both multi-layer folding chirality and orientational chirality. This is indeed the first asymmetric catalytic approach to multi-layer 3D chirality. New chiral catalysts were designed and screened under various catalytic systems that proved chiral amide-phosphines to be more efficient ligands than other candidates. The multi-layer 3D framework was unambiguously determined by X-ray structural analysis showing a parallel pattern of three layers consisting top, middle and bottom aromatic rings. The resulting multi-layer products displayed strong luminescence under UV irradiation and strong aggregation-induced emission (AIE). This work would benefit not only asymmetric chemistry but also materials science, particularly, polarized organic electronics, optoelectrics and photovoltaics in future.
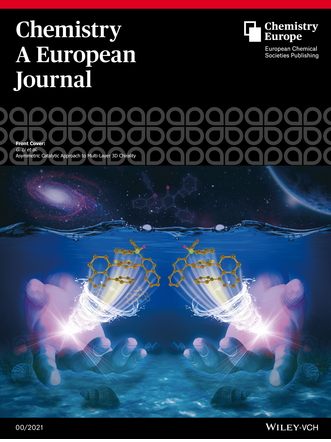
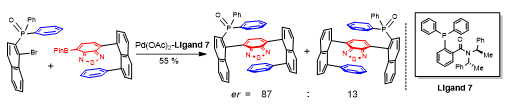
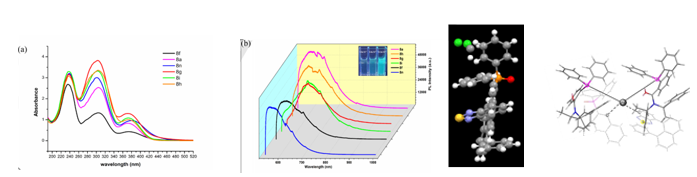
Scheme 3. Asymmetric Single C-C Bond Formation Controls Folding Chirality
4. Asymmetric Catalytic Assembly of Triple-Columned and Multiple-Layered Chiral Folding Polymers
(Chem. Eur. J.https://doi.org/10.1002/chem.202104102; Research, 2022, https://doi.org/10.34133/2022/9847949) (VIP & Front Cover Art)
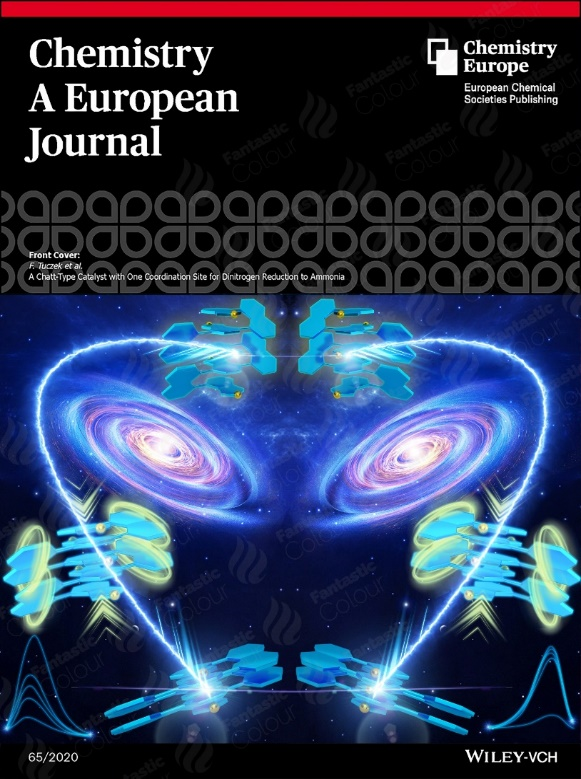
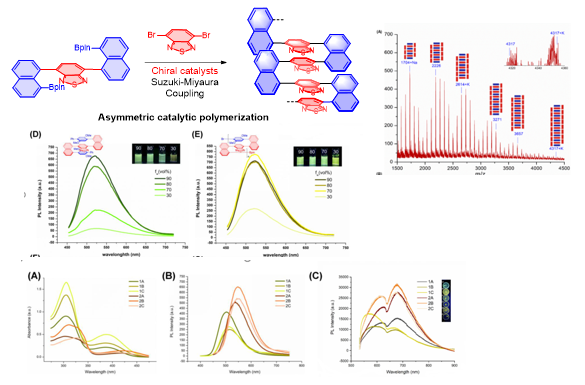
Figure 4. Chiral Folding Polymers and Properties
5. New Catalytic Reactions and Synthetic Light-Harvesting Assembly
(J. Am. Chem. Soc., 2017, 139, 11184; Angew. Chem. Int. Ed., 2020, 59, 3078; J. Am. Chem. Soc., 2021, spotlight paper, https://dx.doi.org/10.1021/jacs.0c12853)
In collaboration with research groups of Prof Ge (Figure 4), Profs Stang and Deria (Figure 5), we conducted C-H activation/funcrionalization and synthetic light-harvesting assembly studies. (a) we achieved Pd(II)-catalyzed γ-C(sp3)–H activation of aliphatic and aromatic hetero aldehydes by using a transient ligand and an external ligand, concurrently. A wide array of γ-arylated aldehydes were readily accessed without pre-installing any internal directing groups. This reaction can be performed on gram-scale and showed its potential applications on the design and synthesis of new mechanofluorochromic materials with blue-shifted mechanochromism properties. (b) we designed and synthesized new trigonal prismatic metallacages of bearing triphenylamine and anthracene moieties to fabricate artificial light-harvesting systems (Figure 6). The new anthracene−triphenylamine chromophore makes possible the tunable excited-state property as a function of the solvent polarity, temperature, and concentration. The synergistic photophysical footprint of these metallacages, defined by their high absorptivity and emission quantum yield (QY) relative to free ligands, signifies them as a superior light sensitizer component in an LHS. In the presence of the fluorescent dye Nile Red (NR) as an energy acceptor, the metallacages display efficient (>93%) excited energy transfer to NR through an apparent static quenching mechanism in viscous dimethyl sulfoxide solvent.
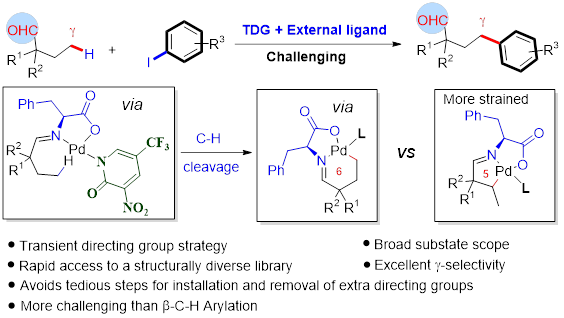
Figure 5. C-H bond activation of aldehydes
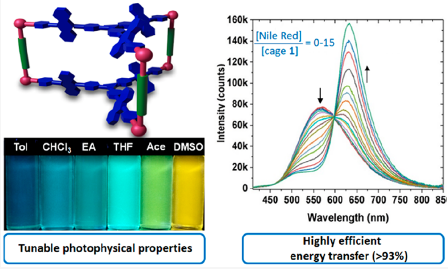
Figure 6. Synthetic light-harvesting assembly for energy transfer
Department of Chemistry & Biochemistry
-
Address
1204 Boston Avenue, Lubbock, TX 79409-1061 -
Phone
806.742.3067